Nano-Optics, Superconductivity and Spin physics Interface
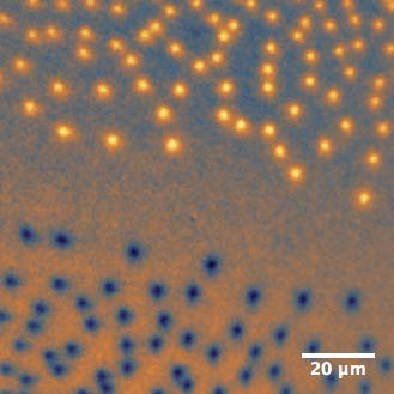
Optical imaging, generation, and manipulation of Abrikosov vortices in superconductors for applications in Josephson transport and single spins control.
The semiconductor electronics scaling roadmap is expected to reach its physical fundamental limits within the next decade. Superconductor electronics is one of the most promising alternatives due to higher operating frequencies, lower switching energies and quantum nature. The realization of new robust platforms becomes essential to herald a new era in technological evolution. We explore and exploit the interplay between nano-optics, magnetism and submicron scale superconductivity in order to decipher fundamental properties of specific superconducting states and to lay the foundation of light operated Josephson transport and nanoscale magnetic manipulation of single spins.
Achievements and Projects
Magnetic field can penetrate into superconductors in the form of Abrikosov vortices, which are magnetic flux tubes surrounded by circulating supercurrents often trapped at defects referred to as pinning sites. With a view to achieving local and precise control of the electronic transport in Josephson junctions, the manipulation and generation of individual Abrikosov vortices in superconductors is appealing. These nanometric-sized objects carrying a flux quantum are the most compact magnetic objects in superconductors. It has been demonstrated that a single vortex placed in the vicinity of a Josephson junction can strongly affect its transport properties through the local supercurrents that induce Josephson phase-shifts. Single vortices can therefore be utilized as flux-quanta bits for the design of high-density digital cryoelectronics.
Single Vortex Magneto-Optical Imaging
Optical manipulation of single Abrikosov Vortices
Handling individual Abrikosov vortices remains challenging. We have introduced a far-field optical method based on local heating of the superconductor with a focused laser beam to realize a fast and precise manipulation of individual vortices, in the same way as with optical tweezers. (Nature Communications 7, 12801 (2016)).
Generation of single flux quanta with Kibble-Zurek mechanism
We have introduced a simple far-field optical method to generate and stabilize single flux quanta at any desired position in a raw superconducting film. It is based on fast quench following the absorption of a tightly focused laser pulse that locally heats the superconductor above its critical temperature. We achieve ex-nihilo creation of a single vortex pinned at the center of the hotspot, while its counterpart opposite flux is trapped at a distance controlled by the pulse energy. The creation of single flux quanta was be performed without the need for any electrical current or external magnetic field, which is the hallmark of the Kibble-Zurek effect (an effect initially introduced in cosmology to explain the spontaneous generation of topological defects in a system submitted to a fast quench) (Nano Lett. 2020, 20, 9, 6488–6493).
Numerical calculation of time-dependent Ginzburg-Landau (TDGL)
In order to model the nucleation of single vortex-antivortex pairs, we have performed numerical simulations of the temporal evolution of the order parameter in the superconducting film, by resolving the time dependent Ginzburg-Landau equations (see Methods in the SI for more details), where the thermal fluctuations inherent to the KZ mechanism are introduced. The movie below displays the spatial distribution of the order parameter modulus and phase at different stages of the quench. Starting from superconductivity destruction by the laser pulse, the simulations provide a qualitative physical picture of the vortex-antivortex pair generation once superconductivity is recovered.
In general, in the Kibble-Zurek mechanism, an equal number of vortices and anti-vortices are produced. We are theoretically exploring the possibility of using the spin and angular momentum of light to promote the generation of vortices of given sign. Under these conditions, one can effectively create a magnetic field in a superconductor with light, which is a hallmark of the inverse Faraday effect.